During the second part of the Infrastructure installment, we will cover some of the more technical elements of aquaculture. This is an arid subject but could be highly valuable for farmers who are starting their business, for those who are looking into modernizing their infrastructure, for those who have high energy costs and would like to do something about it, or in general for all farmers who wish to know more about how their equipment work.
As stated in the first part of the Infrastructure installment, we don’t intend to present all the existing tools to calculate and develop aquaculture infrastructure (we would need to write a book!); we only want to present the basic elements so you can understand and have an informed conversation with the engineer who is in charge of designing your farm. In the case of smaller farms, this could help to get a better notion of your equipment and how to improve it if you are thinking of changing it. In any case, we encourage all farmers to obtain professional advice for any significant investment, such as the acquirement of pumps, aerators, and filtrations systems.
Water pumps and piping
The selection of an adequate pump is crucial for the farm’s success. In several cases, pumps selected by farmers are over-estimated, meaning that they invest in huge pumps with extreme power when in reality, the system could work just as well with something smaller. This is a recurrent problem that is not only negative for the farm’s economic performance but also has a negative impact on the environment. Starting a big pump takes a lot of energy, which is sometimes necessary, but when a case of over-estimation exists, this could result in an unnecessary increase in energy costs, therefore increasing our total production costs and reducing our profitability. Let’s remember that energy is, after the feed, the highest variable cost, and detecting this kind of problem is very difficult if you are not looking in the right place or don’t have the needed knowledge.
As we will mention further, the correct pump choice depends highly on the kind of tubes and pipeline selected for the farm. Different materials have different effects on the energy needed to move water. Also, the width of the pipes, determined by the water flow required in our system, will impact the pump’s power needs. Finally but not less important, the height at which we will pump water and the length of our pipeline will help to finish calculating the pump we need.
Next, we’ll talk broadly on how to select the correct water pump, but in reality, this is one of the most technical aspects of aquaculture, and we suggest a deeper research or reaching out to an expert to evaluate your pumping needs.
First, we need to determine the desired water flow. This is entirely up to the farmer, but there are mainly two elements that can help determine it.
- The water exchange rate. If we intend to perform a 20% water exchange per day, we would need a calculate the required flow simply by obtaining the 20% of our total volume and then dividing it by the number of minutes in a day; that way, we’ll know the volume per minute that we need to be able to pump so that we can maintain this water flow. Using this method, we assume that the pump will be on 100% of the time.
- The fill-up time. This is a significant aspect to consider since longer fill-up times can increase production cycles. Since fixed costs are maintained, this time element increases production costs. For example, if we consider that 20% exchange per day, that same pump would take five days to fill 100% of the ponds, which is a good value; but if we have chosen say a 5% daily exchange rate because we are using aquamimicry systems, fill-up time with that pump would be close to 20 days.
If we must choose between one of these criteria to select a pump, it is best to go with the fill-up time since it will reduce our costs and increase production, even if we have too much power for water exchange.
With the selection of the water flow, we can now estimate the pipe diameter. According to fluid dynamics, four main elements affect the capacity of water to flow through a pipe: the density of the fluid, the speed at which the water flows, the diameter, and the material of the tube.
The density of the fluid could be treated as a constant since we are always working with marine water. Although different salinities and temperatures might change this value, changes won’t be significant enough to change the values obtained. That leaves us with the selection of the material and the diameter.
Again let’s remember a fundamental element of fluid dynamics, the energy conservation principle. This scientific law states that energy is not created nor destroyed, only transformed. Thanks to this, we know that water velocity is lost due to the forces of friction, which will play a fundamental role in our estimations.
Friction is a tangential force on a surface that opposes the sliding of an object across an adjacent surface with which it is in contact. In other words (although not as accurate), and in our case, friction is the energy loss resultant from the contact between water and the tube. With this, we can deduct that:
- As the diameter of the pipe gets wider, energy conservation is superior since there is less friction between the water column and the tube; therefore, less energy is lost, so the bigger the diameter, the better.
- As the material gets smoother (or less porous), the friction contact surface is reduced, increasing energy conservation; hence, the smoother the material, the better.
So, following these principles, we should get the biggest diameter in existence with the smoothest material possible; but as it usually happens in aquaculture, there is one more (very significant) element to consider: costs.
As you have maybe already figured out, if you didn’t already know, as the diameter of a pipe gets bigger, it gets significantly more expensive. For that reason, lots of engineers have come up with several different formulas that allow us to calculate the diameter that minimizes the cost and maximizes the energy conservation. Since other factors than that treated here affect water flow, different formulas have different constraints and implications, so we need to look for the one that best fits our system.
The simplest formula that estimates the best diameter accounting for costs is the Bresse formula, which can be written as follows
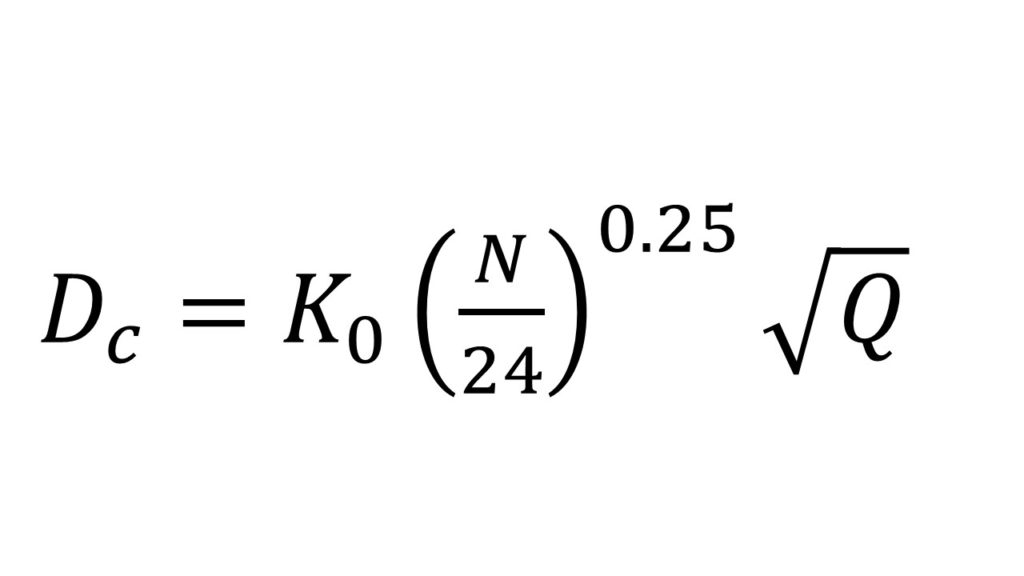
Where K0 is a constant known as the Bresse constant, N is equal to the hours the pump is on during a 24 hour period, and Q is the water flow. This formula has several constraints, making its use highly limited in aquaculture, but it exemplifies the kind of formula that we can use to determine the diameter of our pipeline economically.
Once we have determined the water flow and diameter, we can look into selecting materials. There are charts that will help us observe the different friction coefficients of different materials. In general, the material that has the best smoothness:cost proportion is PVC, which is why it is the most used material for water pipes worldwide. In some cases, where water flow needs to be very high, the diameter needs to be very big, making it impossible to find these PVC pipes commercially. In said cases, it’s common to go for custom-made concrete pipes, especially if they are going directly into the sea since this material can better resist corrosion.
Once the pipe’s material and diameter have been selected, we can estimate the water pump’s power needed to achieve our desired flow. For this, we will need to calculate something called pressure drops (abbreviated as hf), which is basically the estimation of the energy lost through the system; for this, we will need to know the length of the pipeline, all the accessories used (i.e., tees, elbows, valves, etc.), the height at which the water will be elevated, and the speed at which the water moves inside the pipe.
There are a lot of equations to estimate the pressure drops, being the most used one the Darcy–Weisbach equation, which we will not detail, but it will let you know that there exists software that allows to calculate these fairly simply and quickly. With all these calculations, we can finally estimate our pump power requirements using a final formula
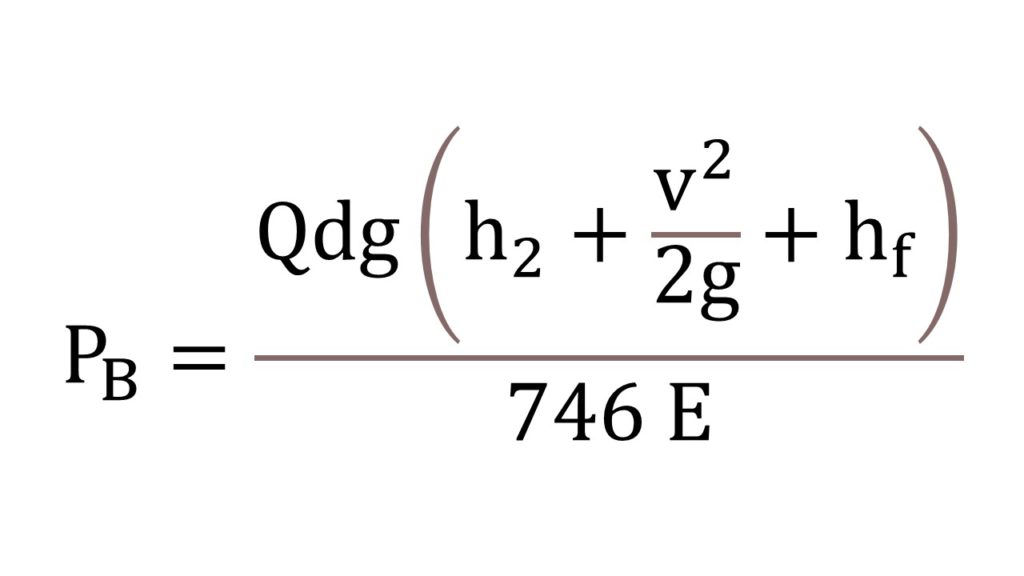
Where, as we saw, Q is the water flow, d is water density, g is the gravitational constant (9.81 m/sec2), E is the pump’s efficiency (if this is not known, one can assume around a 70% efficiency), 746 is the watt-hp relation, h2 is the height at which we will pump our water, the element v is the speed of the water inside the tube, and hf is the value obtained from the Darcy-Weisbach equation, a.k.a. the pressure drop.
Water treatment system
Depending on the system selected for production, water treatment is more or less critical for productivity. The system that needs less water treatment is the production in open systems, usually associated with earth ponds; while the highest water treatment needed corresponds to recirculating systems, where the water used is recirculated into the system; hence the need to treat, filter, disinfect and replenish water quality before inserting it back into the system.
We can divide the water treatment system into five different components: sedimentation, filtration, disinfection, degasification, and oxygenation.
As its name indicates, sedimentation consists of letting the big suspended solids sediment or settle, that is, fall by gravity to the bottom of a tank or settler. For this system to work, the water flow through the settler needs to be as slow and laminar as possible. The design of the settler needs to be correct to work properly. The inflow needs to be set, so the current has a physical barrier with an opening in the bottom, then water can flow up the barrier, it can pass through a granular media or settling media or directly flow through the outflow that needs to be on top of the settler. At the bottom, there must be a slight slope that directs the sediments and sludge to a drain.
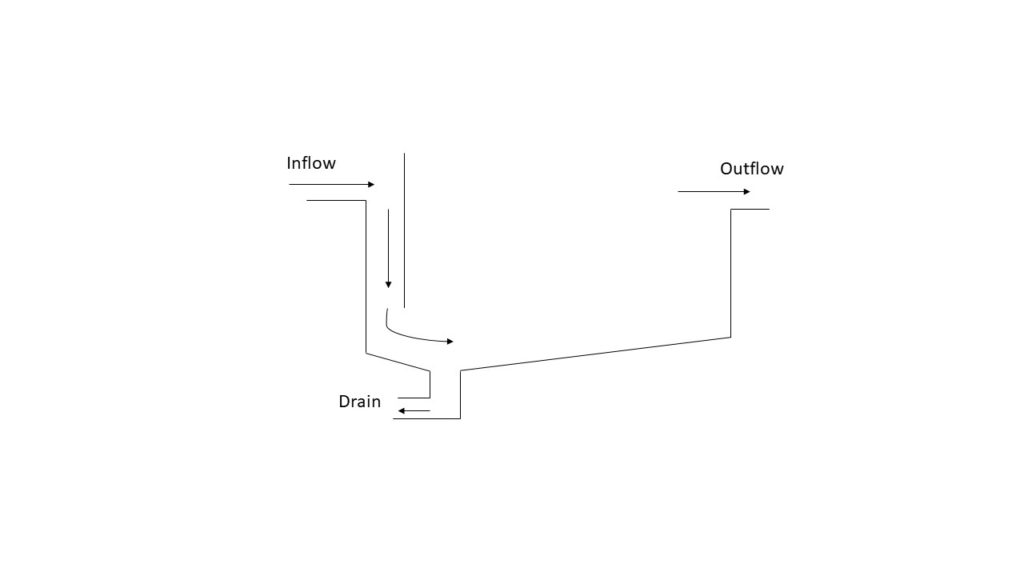
Now, the water is free of big suspended solids, but smaller ones would need too much time to settle, so other methods are used for their removal, mainly mechanical filtration.
Filtration can be divided into several categories; we will talk about the two kinds of filtration systems that are most significant in aquaculture: mechanical and biological.
Mechanical filtration consists in filtering the water through mechanical means, that is, without the use of chemical reactions or living organisms. There are a lot of different kinds of mechanical filters, being the most used ones the sand filters, cartridge filters, drum filters, band filters, and, with a special mention, skimmers.
Band and drum filters work similarly. They use a band that lifts suspended solids from the water and pass through a cleaning area, where the solids are separated, and the water is then returned cleaner. These filters are used for somewhat bigger particles that the settler could not remove; in reality, these filters are not much used in shrimp aquaculture. On the other hand, sand and cartridge filters are plenty used. These kinds of filters work just like the water filters at home; they rely on materials that leave small spaces between them, such as silica sand or cloth with minimal mesh light; the idea behind these filters is to let through the water but retain any small suspended particles.
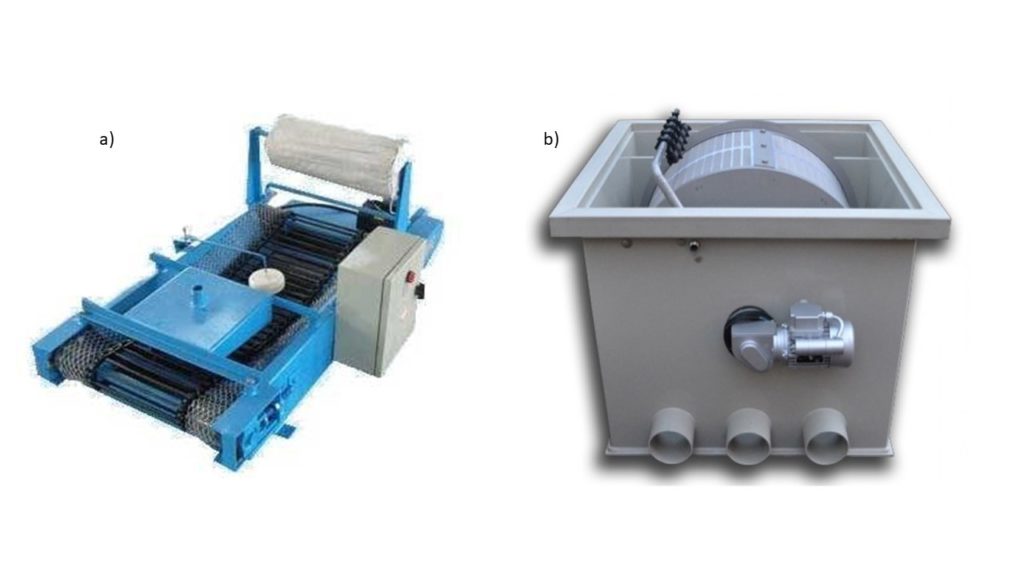
There are pros and cons to sand and cartridge filtration. They both are very effective in separating small suspended particles up to 20 microns, leaving almost anything behind, but smaller (and very problematic) suspended solids, such as bacteria (0.5-0.8 microns for Vibrio alginolyticus) and viruses (0.08-0.12 microns for WSSV). If the water is too charged with suspended solids, these filters will have constant blocks and need periodical cleaning. In that regard, sand filters are superior to cartridge filters since cleaning and maintenance are easier and cheaper in the long run.
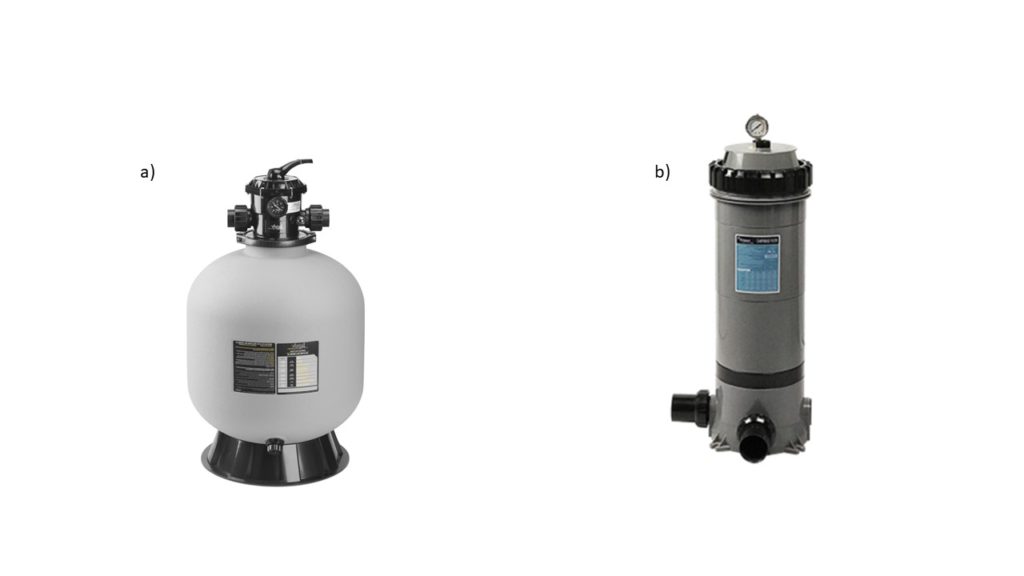
As we mention, the skimmer deserves a special mention. It is a mechanical filter, but it can also function as a disinfection, oxygenation, and degasification system. Therefore, we will discuss it at the end of these lines.
Let’s talk about the other kind of filtration, biological filtration. Let us remember that non-ionized ammonia is highly toxic for fish and shellfish (including shrimp); it is also part of the excretes generated by them, so if we keep high densities in the same water where they eat and excrete, the levels of ammonia will rise. If said water is not treated opportunely, it will severely (and negatively) impact our crop.
Fortunately, there is a way to eliminate ammonia in a non-intrusive and eco-friendly manner, and that is through the use of biofiltration. Biofiltration is nothing but the use of bacteria to eliminate ammonia from the water. These nitrification and denitrification bacteria use a metabolism that relies on ammonia to grow, producing atmospheric nitrogen as a subproduct. The biofiltration then promotes the growth of these bacteria in large containers filled with wrinkly/rough material (the biofilter’s substrate) that increases the surface where they can grow. Then, water with high ammonia concentrations is passed through these containers, letting bacteria use it, and finally, allowing the farmer to collect ammonia-free water. The principle of the biofilter is the same as the in-tank bioremediation or probiotics, but the space where this happens is different, leading to entirely different management systems.
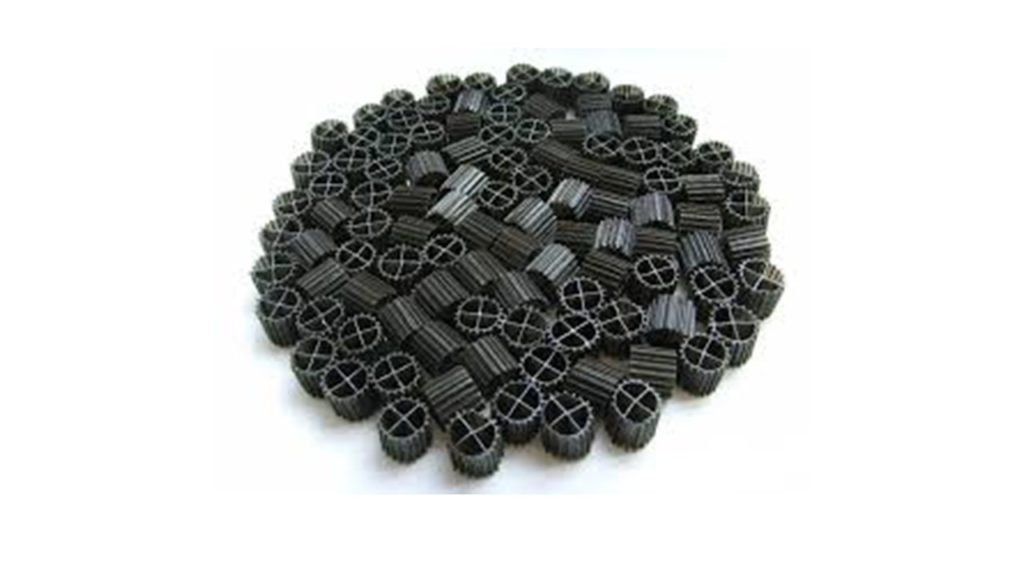
Biofilters can be a little more complicated since one of the metabolic routes followed in the process of eliminating ammonia is aerobic (that means it needs oxygen), and the other one is anaerobic (which means it doesn’t go through in presence of oxygen), so both elements need to be present in a biofilter for it to work correctly.
So now we have water that looks clean, but might still be blooming in pathogen or unwanted living microorganisms in it, so we need to disinfect or sterilize it. There are several ways of doing this, either through physical or chemical means. For the last ones, the farmer needs to be very careful not to use products that might kill or reduce the performance of their shrimp. For example, chlorine is a handy and powerful disinfectant, but it should be used before seeding shrimp and not once they are in the pond.
It is better to use other methods that get the same result but are safer for shrimp and consumers, such as ozone or UV lights for recirculating water treatment.
The installation and use of UV and ozone treatment systems are straightforward and not excessively expensive. In the case of UV sterilization, the filter consists of a tube filled with UV light bulbs; the water is passed through this light with a specific wavelength, killing most living organisms and viruses in the water. UV does this by inserting highly energetic photons in the DNA or RNA of the living organisms, breaking the chemical bonds of these particles, disabling their capacity to survive and/or reproduce. Furthermore, it denaturalizes some proteins, breaking the cell’s wall of bacteria, killing them instantly. Ozone disinfection works similarly, but its energy doesn’t come from photons but the Oxigen free radical present in O3, which is an unstable gas.
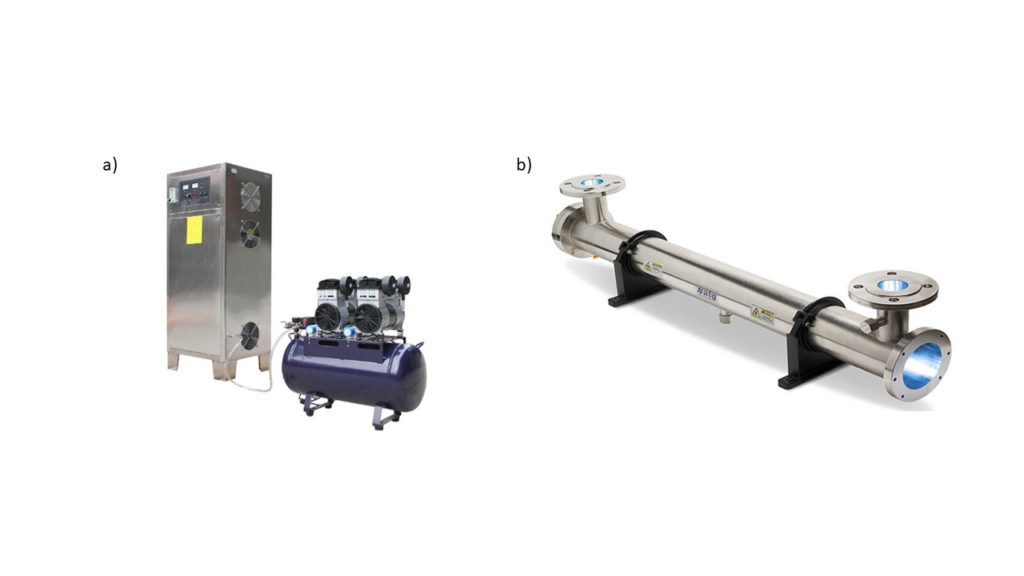
Now the water is free of suspended solids, toxic ammonia, and potential pathogens. But in recirculating systems, there is one more thing to account for: gases.
Just as ammonia is toxic for shrimp, so are high concentrations of CO2. CO2 is not only toxic by itself but is also an acid that reduces the water’s pH, which could lead to reduced productivity and even high mortalities in our system.
Most CO2 present in our water will come from the respiration of living organisms, so high densities will lead to high volumes of CO2. Furthermore, elevated CO2 concentrations in water will reduce the availability of dissolved oxygen, so to solve this, we need to eliminate CO2 from the water. We can achieve this by passing the water through a degasification column or packed column.
A packed column is just a large tube where water is poured. This column is filled with a material that increases the interaction of water and the atmosphere. Due to the different concentrations of gases in the water and the atmosphere, CO2 will leave our treated water, and oxygen will replace it, increasing DO availability.
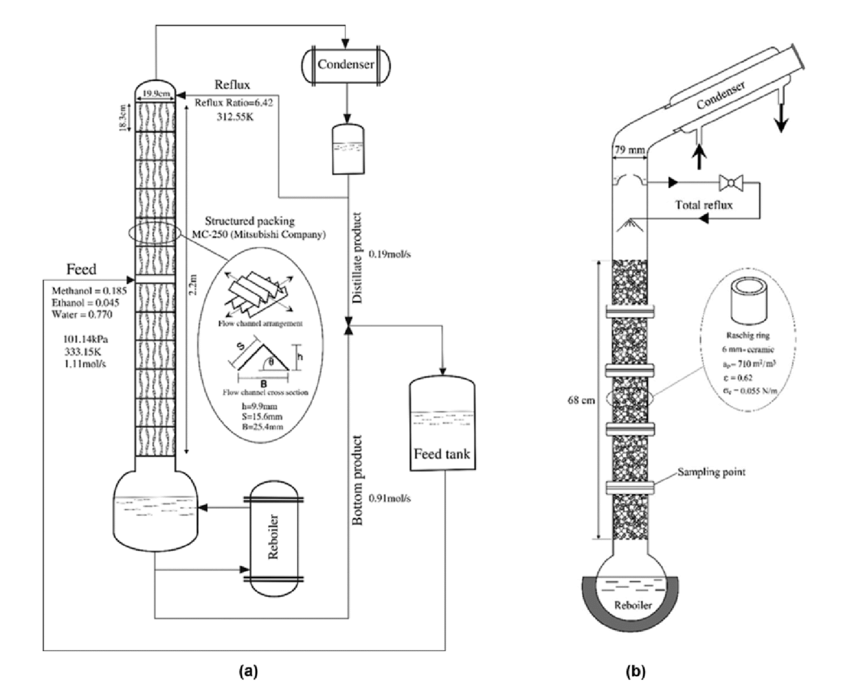
To finalize our cursory review of filters, let’s discuss the skimmer. A skimmer or protein skimmer is a filter that uses air to generate water turbulence. The interaction of air and water in a turbulent manner generates tiny bubbles; these bubbles will interact with water, capturing proteins and small suspended solids and separating them from water as a foam. A skimmer is able to separate very small suspended solids, even some kinds of bacteria. The fact that there is no solid medium makes it easier to maintain and clean and reduces the probability of bacterial growth. Furthermore, the air pumped in the skimmer can be ozone resistant, making it also a disinfection filter. Finally, the interaction of the movement of the water column coupled with the interaction of the interfaces air/water helps to de-gasify and oxygen the water treated.
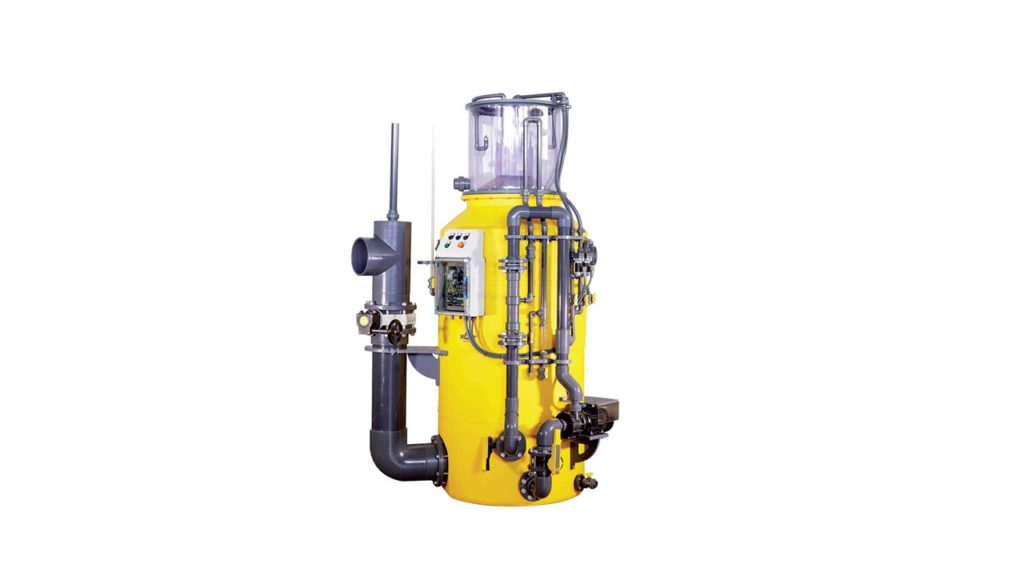
Finally, oxygenation can be achieved by several methods, from the movement of the water column to injection of oxygen; different approaches require different investments and management. We will discuss this further in our next article, which will be dedicated entirely to aeration and water oxygenation.
Remember, not all systems need all equipment. For example, an open system will do just fine with only some sort of mechanical filtration, like a band filter or even a simple mesh; meanwhile, a recirculating system will probably require all of the abovementioned equipment. The size and capacity of each component needs to be adequately calculated, which is why we would like to emphasize the need to hire a professional for the correct size of the equipment.
MUY INTERESANTE, MAS INFORMACION POR FAVOR.